How Lithium Iron Phosphate Batteries Can Help Transform EVs
Lithium Iron Phosphate – enabling the future of individual electric mobility
Today’s ever expanding mobile world would not have been possible without Lithium-ion batteries (LIBs). Developed in the 1990s, they initiated a new age of electric energy storage. Comparatively small batteries allowed the success of mobile electronics like smartphones and wireless devices for the internet of things (IoT). The high production cost in the range of several thousand US$ per kWh up until the early 2000s made LIBs not relevant for large energy storage applications like excess energy storage from the electric grid or e-mobility (1). Progress in production technology and cell chemistry helped to lower the costs for manufacturers. Accompanied by the worldwide effort to reduce the consumption on fossil fuels the LIB chemistry enabled the electrification of billions of passenger and commercial vehicles.
LFP vs. NMC
The main cathode chemistries applied in LIBs were layered metal oxides LiMO2 with M being a mix of nickel, manganese and cobalt (NMC) or nickel, cobalt and aluminium (NCA). The spinel oxide LiMn2O4 (LMO) was also important for many battery chemistries used in electric vehicles. Lithium iron phosphate (LFP) was rarely used in passenger cars before 2020 (2).
The comparison of LFP with NMC 811 in Figure 1 shows that both types of cathode active material shine in different key characteristics. The higher cell voltage, powder density and specific capacity give NMC the clear advantage in volumetric energy density. This is important for long driving ranges that customers know from vehicles with internal combustion engines (e.g. 1,000 km with one tank of gas). The advantages of LFP are longer cycle life, better rate capability and safety. The risk of thermal runaway after a mechanical damage to the cell is much lower. This intrinsic reliability made LFP especially popular for the application in commercial vehicles with frequent access to charging (buses, forklifts, scooters) and stationary energy storage applications often associated to solar parks or wind turbines.
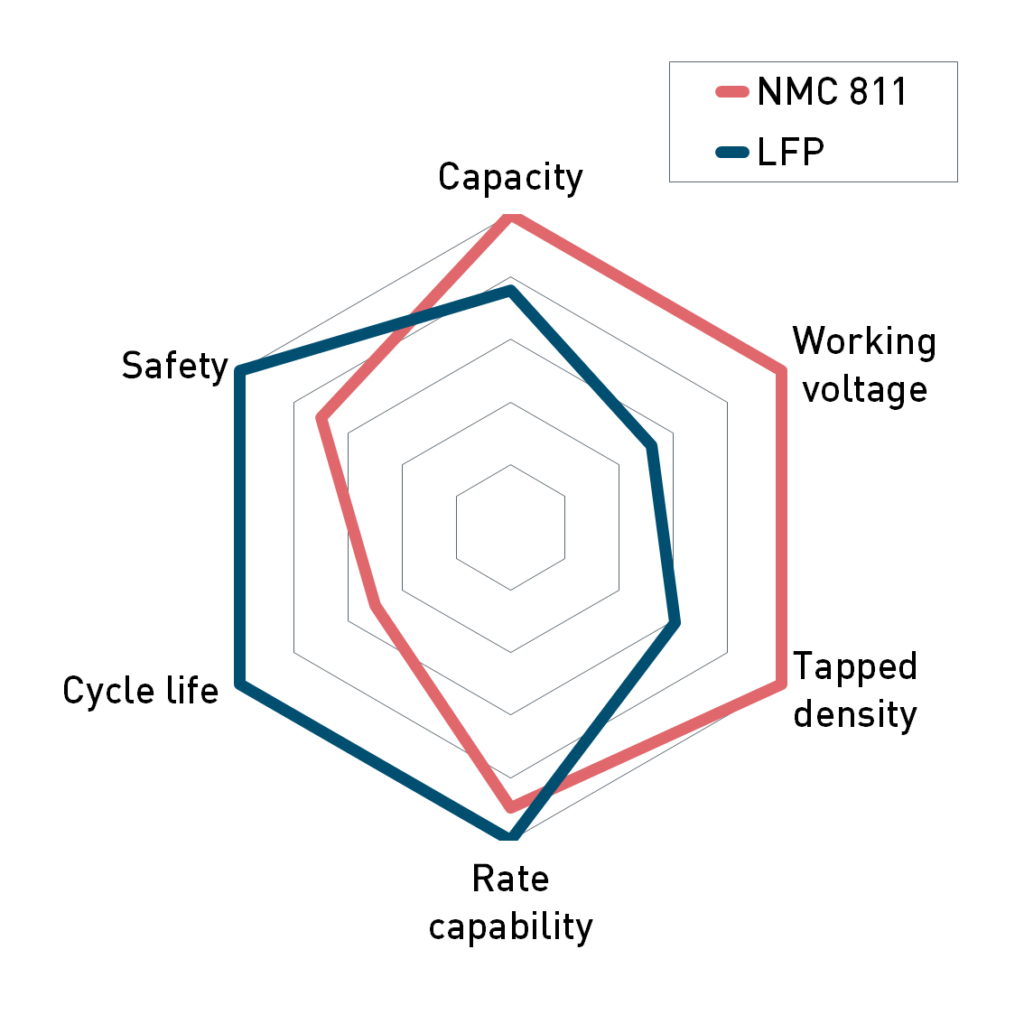
Figure 1: Selected benchmark properties of NMC811 and LFP cathode materials. Data for NMC was adopted from (3).
The growth of electric mobility
Over the past decade, a growing number of governments and car manufacturers announced timelines for the end of the internal combustion engine in the passenger vehicle market and the mass introduction of electric vehicles. In parallel, the entire supply chain announced global investments in production capacity to meet the soaring demand this will cause. For example, the demand for lithium is projected to increase by a factor of 6-7 by the end of this decade (4). This will affect all currently commercialized cell chemistries and lead to raw material shortages as well as sharply increasing prices. Additionally, the production of NMC cathodes requires the critical metal cobalt and also nickel. Mining of both commodities is projected to rise by a factor of 2 by 2030 (4). It is questionable if these increases will be enough to meet rapidly growing demand.
Despite these problems, the first stage of the upcoming electric vehicle era will most likely be set by LIB technologies. There is simply no other energy storage technology that has the technological maturity yet to be employed in the e-mobility sector in the coming years. A mix of cell chemistries will be needed to satisfy the expected demand for batteries – which has brought LFP in the game.
LFP – the backbone of the EV market
For upper class vehicles NMC will continue to be the cathode of choice because of its obvious performance advantages. Smaller vehicles for everyday use, however, do not need the outstanding range of NMC based batteries and can benefit more from the longevity and safety of LFP, which also comes at a significant price advantage. Several major OEMs have already announced to switch to LFP batteries, such as Tesla (5), which will use LFP for their standard range vehicles, as well as the German car manufacturers Volkswagen (6) and Mercedes-Benz (7), which will use LFP for their high volume entry models in the future. This way, LFP will become the bread-and-butter technology of the EV market.
There are several ways to utilize LFP in e-mobility applications (Figure 2). The mixing of cathode materials like LFP and NMC on the cell level can improve the performance compared to the application of cells with only the individual cathode active materials (8). A mix of single-chemistry modules can be useful for battery management to improve the low temperature range performance (9).
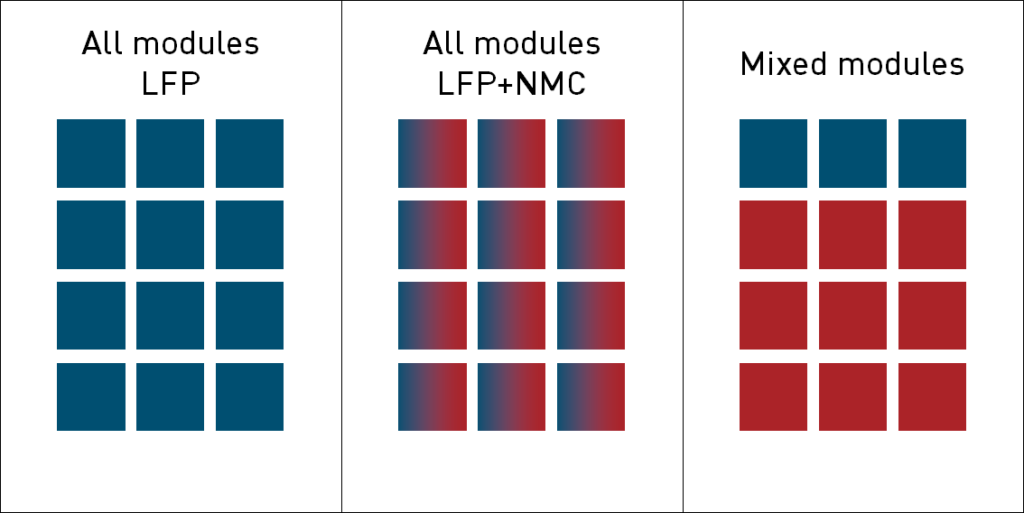
Figure 2: Concepts to incorporate LFP into a vehicle battery pack.
The ongoing development of new battery materials will lead to a shift in the electrochemistry landscape. Improvements on LIB chemistry are aiming towards high nickel NMC materials and LMFP (lithium manganese iron phosphate). The substitution of iron with manganese can provide phosphate based cathodes with higher volumetric energy densities to further establish these materials in the price sensitive high volume market segments. Alternatives like all solid state batteries or sodium-ion chemistry will be options in the future.
Getting the most out of LFP
At IBU-tec advanced materials, we are currently looking into improvements of the particle composition. Based in Germany, IBU-tec is currently the only LFP manufacturer with an established production site in Europe. The company has been involved in the research and development of LFP cathode material for over 10 years. Since the third quarter of 2021 its phosphate cathode materials are sold under the product family name IBUvolt.
One of these is the power-grade product IBUvolt LFP400, which was particularly developed for special purpose applications in passenger vehicles, stationary energy storage and maritime environments. Compared with other LFP grades available on the market, IBUvolt LFP400 has a significantly higher carbon content. This leads to a much better conductivity and, in result, to a low direct current resistance. At 5C charging rate a state of charge (SOC) of more than 80% can be achieved (Figure 3). This positions the material well for fast charging applications. Especially for vehicle batteries relying on LFP for economic reasons the ability of fast charging can compensate the lower range compared to batteries with higher energy density. As a component of a battery pack LFP cathodes can support other high performance cell chemistries to combine the advantages of the materials.
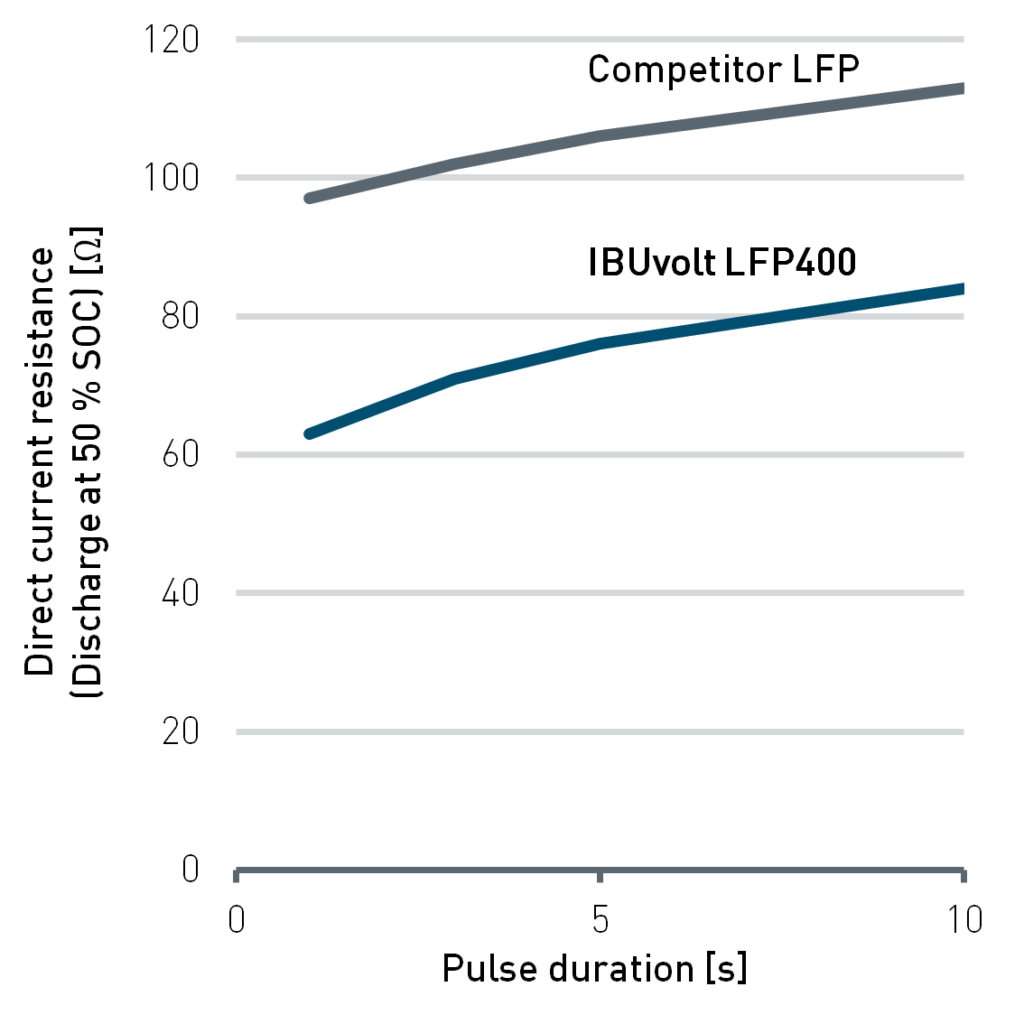
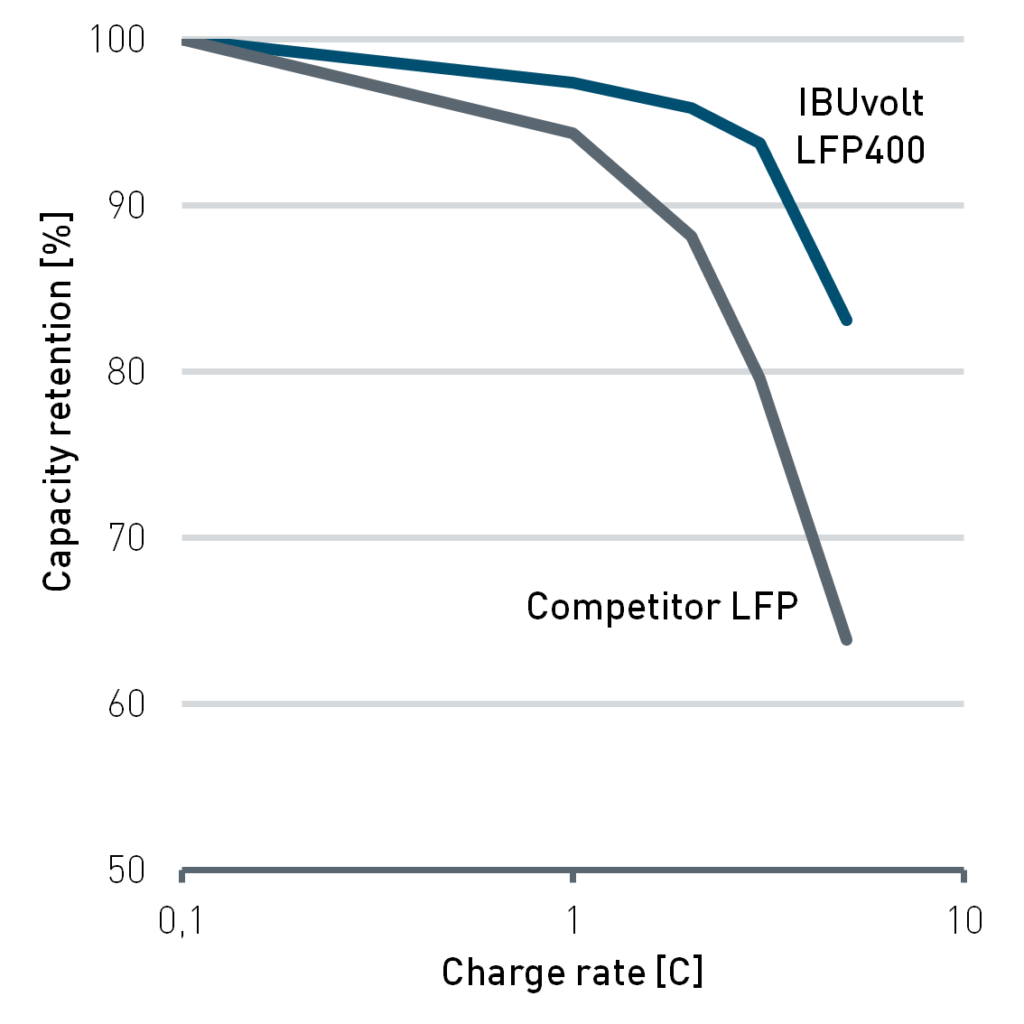
Figure 3: Comparison of direct current resistance (left) and capacity retention during charging (right) for IBUvolt LFP400 and a competitive LFP product. Testing was performed in pouch cells at 25 °C with graphite anodes. Cycling was performed from 2-4 V.
In addition, the high carbon LFP chemistry of IBUvolt LFP400 results in a remarkably high cycle life. Cycling against a lithium titanate (LTO) anode at 2C results in more than 90% capacity retention after 9,000 cycles (Figure 4). A cathode with this LFP material will be the longest lasting component of any vehicle, with second life applications well within its expected lifecycle. This reduces the material stream going towards recycling. Therefore, the already low ecological footprint of LFP is further reduced. The longevity of LFP also lowers the total cost of operation for the battery compared to NMC.
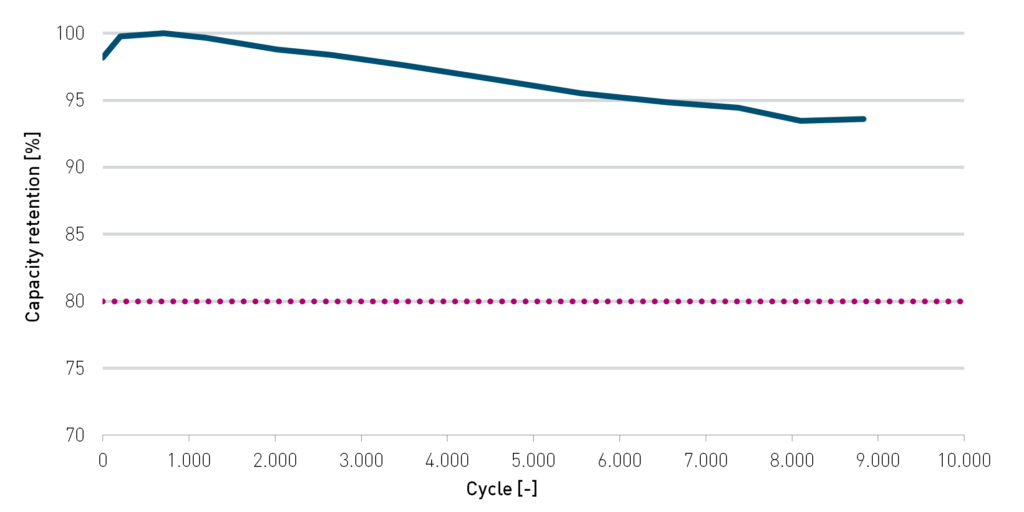
Figure 4: Cycle life of IBUvolt LFP400 with 80 A current (2C charge/discharge). Full cell with LTO anode and proprietary electrolyte.
Summary
LFP batteries have become more and more important for e-mobility applications. The cell chemistry has certain advantages in some use cases over more expensive and critical NMC materials. Ongoing developments are made to improve the conductivity and energy density of LFP cells. IBU-tec is at the forefront in research and production of LFP in Europe and has achieved very good results with a high carbon power-grade LFP cathode material and started to develop also LMFP material. In the short to mid-term, LFP will be the backbone of the e-mobility revolution, leading to further improvements in battery technology.
Dr. Stefan Schwarz, IBU-tec advanced materials AG
1. Re-examining rates of lithium-ion battery technology improvement and cost decline. Ziegler, Micah S. 2021, Energy Environ. Sci., pp. 14, 1635-1651.
2. Performance and cost of materials for lithium-based rechargeable automotive batteries. Schmuch, Richard. 2018, Nat. Energy, pp. 3, 267-278.
3. Long-Term Cyclability of NCM-811 at High Voltages in Lithium-Ion Batteries: an In-Depth Diagnostic Study. Li, Wangda. 2020, Chem. Mater., pp. 32, 7796-7804.
4. World Economic Forum. A Vision for a Sustainable Battery Value Chain in 2030. 2019.
5. Tesla Investor Relations . Quaterly disclosure. [Online] Q3 2021. https://ir.tesla.com/#tab-quarterly-disclosure.
6. Volkswagen Power Day. [Online] March 15, 2021. https://www.volkswagenag.com/de/events/2021/Volkswagen_Power_Day.html.
7. Bloomberg. [Online] October 27, 2021. https://www.bloomberg.com/news/articles/2021-10-27/mercedes-bets-entry-level-ev-buyers-will-accept-shorter-range.
8. A review of blended cathode materials for use in Li-ion batteries. Chikkannanavar, Satishkumar B. 2014, J. Power Sources, pp. 248, 91-100.
9. NIO. Newsroom. [Online] September 23, 2021. https://www.nio.com/news/nio-launches-standard-range-hybrid-cell-battery.
10. Wood Mackenzie. LFP to overtake NMC as dominant stationary storage chemistry by 2030. [Online] August 17, 2020. https://www.woodmac.com/press-releases/lfp-to-overtake-nmc-as-dominant-stationary-storage-chemistry-by-2030/.